Integrating engineering systems into the master plan
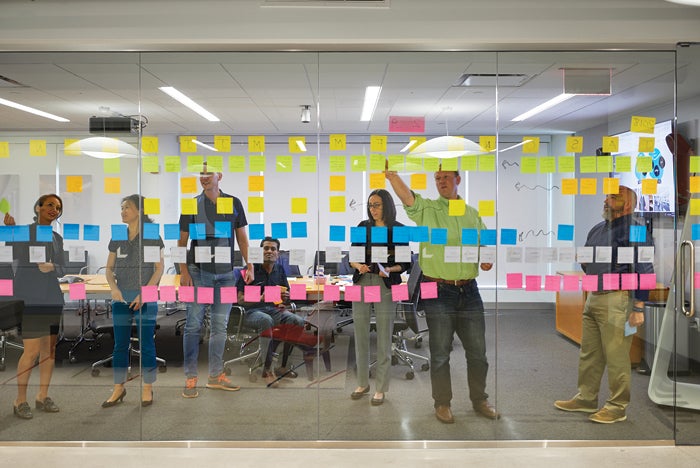
A health facility project team creating a pull schedule.
Images courtesy of HGA Architects and Engineers
When new buildings are proposed or building expansions are planned, a health care organization often engages an architectural firm to assist in creating a master plan to implement the current and future needs as well as the vision of the organization.
A complete master plan that fulfills the “triple aim” of improving the patient experience of care, improving the health of populations and reducing the per capita cost of health care must include examination and consideration of the detailed engineering system needs.
This is even more important given the enhanced requirements of emergency preparedness to address not only day-to-day operations but also natural and manmade disasters.
Understanding the type of engineering systems necessary to sustain the facility functional and performance goals helps inform space requirements and adjacencies for the engineering systems to maximize system effectiveness and minimize system operational and capital costs.
This effort progresses on a parallel path to the programming, operational and master planning steps of predesign.
The current state
It is important to identify the current state of the project before the future state can be achieved. Whether the project is an addition or replacement, there is immense value in understanding the perspective from which the client and their staff is starting.
Working to understand the current state helps to build empathy between the design team and client users and enhances trust early in the project.
Through this process, the engineering team familiarizes themselves with the existing setting, gets leveled in project parameters such as program, schedule, phasing and budget, and asks focused questions to understand the health care organization’s goals and aspirations.
This approach helps to gain consensus of the future state “wants” for the project.
Knowing where the organization starts and where it wants to end up, combined with the desire for innovation in project design, lends itself well to an integrated master planning process. Setting realistic and achievable goals at the start of a project process sets the stage for a successful outcome.
The way to achieve this is by including all impacted team members, which allows the project to be viewed from many different vantage points. This effort will then shape the project priorities and goals.
Benchmarking the best
Innovation and creativity are great if they can be feasibly implemented — and the engineering team is key to the process, with all of the appropriate stakeholders taking an active role to create buy-in for the final outcome.
Finding the right answer is never an easy or direct endeavor, but it can be achieved through a thoughtful design process that begins with benchmarking best practice design solutions for each building system. This approach seeks to ground all team members in what’s possible today as a starting point from which to innovate toward stretch goals and align scope, quality and budget. Best practice considerations will include factors such as energy efficiency, sustainability, reliability, maintainability and resiliency goals.
The result of this effort is documented as the owner’s project requirements (OPR), a tool that the commissioning industry uses as a way of verifying that the installed infrastructure systems meet the owner’s intent. By reviewing energy efficiency, sustainability, reliability, maintenance and resiliency goals at the start of the project, the entire team can understand the objectives and how they affect the master plan.
For example, ensuring that the mechanical systems are reliable and resilient may necessitate modules of mechanical equipment, with an extra or N+1 module to allow for full capacity with one module inoperative. As a direct consequence, the area and cost allocated for the mechanical plant will increase. A robust discussion about infrastructure needs versus wants takes on more urgency when faced with the realities of cost and space. Following are some benchmark considerations:
Energy performance. Fulfilling the aim of being operationally efficient through a high-performing building starts with the project’s overall energy goals. For a recent project in the Midwest, the client had a robust energy tracking system and good data to show an annual energy use of 225 kilo-British thermal units per square foot per year (kbtu/sf/year). After benchmarking the existing facility against comparable facilities, new buildings and code requirements, the challenge for the patient tower addition was set to reduce the energy use to below 150 kbtuh/sf/year, while providing for a resilient, redundant heating and cooling plant.
The value of this benchmarking approach was as follows:
- A clearly defined energy goal early enough in the project to influence master planning.
- A unified team around a common goal that led to enhanced collaboration in design.
- A commitment to innovate toward meeting the goals and defining the new best practice for the facility.
Emergency operations planning. When engaged in the master planning process, it is necessary to understand the codes, guidelines and regulatory requirements that are applicable to the facility. These may vary by location and type of facility, so it is important to understand the requirements specific to the project before getting too far into the planning process.
Almost as important as the functional program requirements is knowing the facility’s emergency operations plan and how it will be implemented or modified for the new scope of work. The Centers for Medicare & Medicaid Services (CMS), National Fire Protection Association (NFPA) and the Facility Guidelines Institute Guidelines are all strengthening the requirements and criteria for emergency preparedness for health facilities, so understanding this is important not only for design but also for compliance.
Defining the codes and guideline requirements while working with the facility to understand their hazard vulnerability analysis (HVA) will inform their emergency operations plan and allow the facility to create a complete picture of how the building will function in an emergency. Chapter 12 of NFPA 99, Health Care Facilities Code, along with the 2016 CMS final rule on Emergency Preparedness Requirements for Medicare and Medicaid Participating Providers and Suppliers, provide criteria for creating the emergency management plan.
The facility is required to have a documented plan not only to address staff and clinical roles, mitigation, communications and safety, but also to address the requirements for the continuity of operation for engineering systems. With a documented plan in place, the physical environment and engineering systems can then be evaluated and designed to support the plan.
By bringing together the same people who create the emergency operations plan to provide input to the master planning efforts, project leaders can assure alignment with the facilities’ goals. These decisions will become the basis of design for the architecture and the engineering systems and will be valuable in implementing the next steps to accomplish the desired resiliency in the systems.
This, in turn, will help inform the size and location of the engineering spaces required, and the level of redundancy necessary to sustain the plan. By understanding this criterion during the master planning phase, the programming, along with the engineering support spaces, can be consistent with the everyday needs as well as the needs of an emergent event.
Emergency backup systems. The essential electrical systems of a health care facility are also significant design features considered through benchmarking and the OPR process that have a functional, space and financial impact. Chapter 6 of NFPA 99, Health Care Facilities Code, and Article 517 of NFPA 70®, National Electrical Code®, indicate loads that are required to be supplied by the essential systems branches.
The life safety branch, which supplies power to loads for fire alarm, generator set support equipment, emergency communication instructions and building egress, is the most limiting branch of the essential systems and does not allow any additional loads. The critical branch is the second branch of the essential electrical system and supplies power for a designated list of direct patient care loads but also allows “additional task illumination, receptacles, and select power circuits needed for effective facility operation.” Equipment branch power also has a defined list of loads that are required, but it too also allows for “other selected equipment.” And then there are the “optional” loads that are not code required but are allowed on the generator (or alternate power source) distribution as long as they do not compromise the code-required essential electrical loads.
The OPR process utilizes the functional program, the HVA and the emergency operations plan to determine the level of essential systems desired for the facility and, depending upon the choices, the load can range anywhere from code required minimum to the entire facility on backup generation.
Ideal state solution
With the team aligned around common performance goals, the task is now to craft a holistic master plan that optimizes solutions for experience, patient outcomes and efficient operations. This requires intense collaboration among architects, engineers, estimators, contracting partners and owners to achieve the ideal state solution.
For the Midwestern project mentioned previously, the team used a set-based design approach that considered first costs, durability, serviceability and long-term operating costs as key criteria in the decision-making process for engineering systems.
After a thorough review, the team chose to concentrate on reducing energy through system static pressure reduction, using heat recovery chillers and a 130-degree hydronic heating system, by recovering energy from exhaust and relief air streams, and by monitoring the energy use of the campus as a whole, with a control sequence that optimizes energy use.
To reduce static pressure, the air-handling units and large ductwork near the air-handling units and shafts were designed for lower than typical air velocity, taking advantage of the fan laws that indicate that fan horsepower (i.e., energy) changes at the cube power of velocity. The 130-degree hydronic heating system is needed because heat recovery chillers can simultaneously provide chilled water and heating water up to 130 degrees, meaning that the hydronic heating water is “free” when there is a simultaneous need for both heating and cooling.
Providing coils in the exhaust and relief air streams captures energy from the conditioned space of the hospital before it is directed to outside. And one of the larger energy savers is the ability to optimize the campus energy use. It turns out that there are many hours where it is more efficient to load the heat recovery chillers and obtain “free” hydronic heating water than to maximize the “free” cooling at the air handling unit using the economizer dampers.
Together, these strategies helped the hospital meet its aggressive energy use goals and a master plan ideal state that optimized the totality of the project.
Decision integration
Understanding engineering systems choices and how they affect space requirements and project cost also is an important aspect of the master planning effort. The engineering team needs to be able to provide a high-level summary of the pros and cons of the systems choice, and how the choice impacts both the master planning effort as well as long-term characteristics such as energy savings, maintenance and ability to meet regulatory requirements for the built environment.
For example, during the OPR discussion for a patient tower, the team may discuss the use of centralized versus floor-by-floor air-handling units. This has a significant effect on the master planning effort as the centralized system needs a large air-handling unit room and large shafts running vertically through the building, while the floor-by-floor air-handling units require multiple, smaller air-handling unit rooms located on each floor, with smaller shafts.
This decision also impacts other engineering systems such as how the electrical distribution is configured and essential systems power is distributed. The team needs to be able to describe the differences in maintenance, infection control, noise control, energy efficiency, redundancy and future flexibility for all the system options.
Because the systems have drastically different impacts on the floor plans and the long-term use of the patient tower, it is not possible to postpone the decision regarding which engineering system to use until later in the design process and should be part of the master planning effort. Following are some tools and strategies:
A3 decision making. During the master planning effort, there typically isn’t time, nor is it warranted, to perform a full life cycle cost analysis for each contemplated option. This could be done during later phases of the project. What is needed during master planning is a library of systems and their characteristics that can quickly be modified and adjusted for the specific circumstances related to the master planning effort.
One way of doing this is by creating an A3, which is a term borrowed from Lean processes. An A3 is a problem-solving tool — literally an 11-inch by 17-inch sheet of paper — that summarizes the current state of the problem, analyzes the situation, documents options, defines success criteria, studies the options, evaluates financial impact and provides a recommendation. The value of the A3 is its brevity and consistent approach. It also can be used to convey technical information to nontechnical team members, and it is an easy method to retrieve the information used to make decisions.
Set-based design. A3s are often used in conjunction with a set log. A set log is a simple matrix that compares multiple system options against the master planning priorities, like first cost, energy cost, maintenance, future flexibility, infection control and noise control. The design team uses its library of A3s to choose four or five system options, compares the options against the priorities, and reviews the set log with the decision-making team.
There are attributes of the set log that make it good for group decision making. One is that the system choices can be represented by a graphic, either of a system or key component of the system. Using a visual reminder of each system helps keep the choices clear.
Another way to assist decision making is to color code the results, in a “good, better, best” manner, making it easier to see which systems meet more of the project priorities. This tool isn’t as accurate as a life cycle cost comparison but is useful for narrowing the choices during the master planning process.
Target value design. With so many project objectives to fulfill within the constraints of a fixed budget, it’s important to have a disciplined process that optimizes the whole and aligns goals and decision making with cost. Target value design, or “design to budget,” is a process model adapted from Toyota’s knowledge-based design methodology that creates the cost context in which final decision making occurs. This approach organizes the project into interdisciplinary component teams and provides each with a target budget to which they are responsible for designing.
Typical teams are site, structural, envelope, interior, thermal comfort, power and technology. Each team is responsible for developing solutions that respect design goals and the budget parameters. The teams use real-time estimating and constant constructability reviews to alignment with objectives. This approach ensures that quality is maintained consistently across all aspects of the project within the budget, while allowing for adjustment for specific project priorities.
Facility-specific design
By engaging all the stakeholders at the inception of master planning effort, systems and space allocations can be more thoughtfully planned and located.
Integrating engineering requirements into a facility master plan results in a resilient, energy-efficient and effective building solution to create an innovative and facility-specific design.
Krista McDonald Biason is senior electrical engineer, Mark Bultman is health care principle and Jeff W. Harris is director of engineering at HGA Architects and Engineers. They can be reached at KBiason@hga.com, MBultman@hga.com and JWHarris@hga.com.