Designing clinical labs
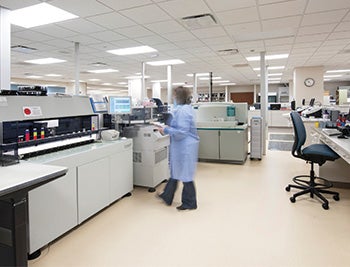
Automated laboratory lines have streamlined lab processes, allowed for better staff allocation, boosted productivity and reduced errors and cost at Parkview Regional Medical Center in Fort Wayne, Ind.
While clinical laboratories are among the most important spaces hospitals and related health care facilities may operate, their functional designs are sometimes less noticed than those of higher profile areas.
However, technological breakthroughs that are changing the way clinical laboratories function are attracting the attention of the health care industry’s top designers as they help these spaces to play an important role in improving the flow of staff and specimens.
Four major changes
Key components of these breakthroughs are four major changes that are contributing to improved clinical laboratory efficiencies and helping hospitals to answer new challenges.
You may also like |
Ten steps to an efficient lab layout |
Research hospital design boosts efficiency |
|
As lab work continues to move away from manual bench testing to increasingly more automated processes, for instance, open-plan designs are providing the flexibility necessary for labs to easily add analyzers or adapt to provide more efficient workflows.
Likewise, as attention to possible threats from new and emerging infectious diseases and bioterrorism increases, designs with the architecture and engineering features needed to isolate and safely handle biohazardous materials are more important than ever, facilitating a rise in the construction of Biosafety Level 3 (BSL-3) containment environments.
And, with the emergence of molecular testing as a new tool for diagnosing and treating disease, labs are being designed to prevent the contamination of specimens used in this type of analysis.
1 Open-plan designs. In the past, clinical laboratories were compartmentalized. Labs were designed for manual bench testing, with techs working in separate, walled-off environments. Many hospital labs are now struggling with the physical layout of their spaces. Across the United States, hospitals with older labs are faced with the prospect of tearing down walls or built-in cabinetry to expand operations or add new equipment.
The latest lab designs feature wide-open spaces. With the open plan or big room concept, a lab is built with no interior walls to allow the layout to be reconfigured as necessary. Power, data and gases are mounted overhead, rather than provided through the floor or walls. Modular casework, which often is equipped with wheels for easy relocation, is used in place of fixed cabinetry. Sinks and floor drains, which cannot be moved without major construction, are placed in areas that are unlikely to change, such as aisle walkways. Drains also can be installed in a regular grid formation throughout the lab, to be capped or uncapped as needed. This arrangement is especially useful with analyzers that require a deionized water feed and need access to a nearby drain to discharge wastewater.
Using an open-plan design, a lab director and technician could reconfigure a portion of the lab themselves over a weekend to add an analyzer and be up and running for testing on Monday. There’s no need to engage a contractor or the hospital’s construction services department to put up temporary barriers, cut down gypsum board and stud walls, or reroute plumbing. Hospitals don’t have to develop phasing plans or suspend operations to update lab workspace.
Many hospital clinical labs are adopting Lean operational models to prevent errors and wasted movement, wasted space, wasted energy and wasted time. Open-plan labs help to facilitate the implementation and continued operational goals of Lean design.
Open-plan lab design and movable casework are also useful for new instrument validation. Before making the final decision to buy a new analyzer or change vendors, the lab can bring in an analyzer for a “test drive” or to benchmark its performance against existing equipment, without worrying about where the instrument under consideration can fit into the lab.
For instance, the lab at Huntsville (Ala.) Hospital, which opened last fall, covers 30,800 sq. ft. over two floors. The main floor, which houses all of the 24-hour and STAT testing departments, including the core lab, microbiology and blood bank, has an approximately 12,000-sq. ft. floor plate. In this area, the only fixed building features are the structural columns needed to support the roof. The floor drains are installed along a 16-foot grid so that no one location is more than 8 feet from a drain; cover plates are used to cap off drains that are not in use. On average, the hospital lab needs to update analyzers or the physical layout of the space at least every three years; this design is intended to maximize long-term flexibility by minimizing the amount of work required by a contractor to rearrange the space.
Likewise, Northern Colorado Medical Center (NCMC) in Greeley, part of the Phoenix-based Banner Health system, opened a new 14,495-sq. ft. open-plan lab last year. The hospital’s previous lab, which was more than 30 years old, was compartmentalized, with high cabinetry that made it difficult to see more than 5 feet across in several areas. Matt Hailey, NCMC director of laboratory services, Banner Health, says the design interfered with communication among techs. The new layout provides the flexibility to add or adjust the lab’s services, platforms or test menu in the future, as well as the ability to cross-train staff members to operate more efficiently today.
2 Automated testing. Automated testing systems, an advancing lab technology, is facilitated by open-plan lab design. In an automated system, analyzers are arranged along a robotic track that operates much like a conveyor belt. Depending on the number of analyzers, automated systems may involve 50 to 60 feet of linear track bolted to the floor. The automation line functions as the backbone of the lab. The line should be located where it won’t act as a barrier or bisect any traffic or workflow to other, more manual testing areas of the lab. In addition, the front end, or pre-analytic, section of the line should be placed as close as possible to where specimens come into the lab. Ideally, a specimen processor sitting next to the lab’s pneumatic tube station will be able to take specimens out of the tube and simply turn his or her chair to drop the specimens directly into the inlet of the automated testing line, with no wasted movement or time.
Because most automated lab systems are scalable, they function well in labs with open-plan designs. A lab can start, for example, with two chemistry analyzers; in a few years, the lab could easily extend the line to include a third analyzer. Or the lab could replace an analyzer with one that can perform a higher number of tests per hour. The open-plan design allows for modular growth.
For instance, when NCMC opened its new lab last year, the hospital went from stand-alone analyzers to a full-blown automated line. NCMC handles all the STAT testing for its own emergency department and Level II trauma center, plus outreach testing for several other Banner Health facilities in the region.
Since automating, NCMC’s volume of billable tests has risen from 610,346 to 1,017,244. Cost and productivity metrics, as measured by Chi Solutions Inc., Ann Arbor, Mich., also have improved. Compared with national peer group benchmarks, the lab has moved from the 77th to the 87th percentile for total cost per test, from the 66th to the 89th percentile for labor cost per test, and from the 86th to the 97th percentile for the number of tests per worked hour with no additional staff.
3 Biosafety Level 3. Lab design is also being affected by a resurgence in BSL-3 containment. Traditionally, this level of containment has been the province of large academic medical centers and military facilities. Today, with increased concern about infectious disease following outbreaks of severe acute respiratory syndrome, swine flu, bird flu, Ebola and Middle East Respiratory Syndrome, as well as increased concern about bioterrorism, even small community hospitals are becoming interested in providing this level of protection in the lab to ensure safe handling of specimens, even if only to send them out for further testing.
McKee Medical Center, Loveland, Colo., also a member of Banner Health, recently installed a small BSL-3 lab. The hospital does not plan to perform identification testing or culture specimens in-house but wants to be able to safely manage suspected dangerous pathogens such as Ebola, anthrax or multidrug-resistant tuberculosis.
BSL-3 labs require specialty design and construction. This includes nonporous materials, so pathogens cannot, for example, get into the plywood substrate of a countertop and multiply. Hardened epoxy-coated or protected walls are necessary so scratches in paint can’t present a similar hazard. Plumbing and vacuum lines must be fitted with multiple vacuum breakers and all penetrations into the room must be sealed so that pathogens cannot escape in the event of a loss of pressure. All plumbing leaving these areas should be either thermally or chemically disinfected. Pass-through sterilizers/autoclaves are recommended between the testing room and the anteroom, so that everything is run through a terminal sterilizer before being thrown into the biohazardous trash.
Specialized standard operating procedures and additional training are essential to the safe operation of a BSL-3 lab, but proper design is just as important. The architecture and mechanical and plumbing systems have to be designed appropriately to ensure safety.
4 Molecular testing. Another recent medical advance that’s influencing the design of clinical labs is molecular testing. Molecular diagnostics and testing quickly are replacing traditional microbiology and are the fastest-growing areas of the clinical lab. Molecular testing is an essential component of personalized medicine, which is based on a patient’s individual genetic makeup.
With molecular testing, lab techs can, for example, directly test the blood of a patient with a suspected systemic blood infection. It’s not necessary to incubate a specimen for a lengthy period. Directly testing a blood sample for DNA markers from specific organisms can produce results in an afternoon, rather than overnight or over several days. Based on specific genetic markers in the sample, techs can determine what type of bacteria, virus or parasite is present and whether or not it’s carrying, say, the genes for amoxicillin resistance.
Molecular testing also can be used to identify cancer markers in tissue samples. Instead of sending a sample of a suspected cancer to an anatomical pathology lab for overnight tissue processing and review by a pathologist, the tissue can be tested directly for specific genetic mutations that can reveal exactly what type of tumor is present. The tumor then can be treated using the best chemotherapy for that type of mutation; a database matching cancers to effective chemotherapies is under development.
Pharmacogenomics is another promising area for molecular testing. Some people metabolize medicines differently than others, due to their genetic makeup. A medicine may work for one patient but not another, or may present negative side effects, based on an individual’s genes. Molecular testing can help to establish, for example, whether a patient would be better served by chemotherapy or an immunotherapy treatment. This is medicine targeted at a specific patient’s immune system, body chemistry or condition.
Large volumes of specimens aren’t needed for molecular testing. Specimens are amplified during the process so that certain genes or portions of DNA that normally occur in small numbers are multiplied up to tens of millions of times. To avoid contaminating specimens — and then amplifying that contamination — a controlled lab environment is needed. The front-end extraction and amplification process requires a specific type of environment and specific unidirectional workflow to avoid contamination.
Simply converting an old manager’s office into an extraction room and a storage room halfway across the lab to be the amplification room won’t work; a specimen or tech should never pass back through an area until after the specimen has been amplified and stabilized. Extraction and amplification rooms should be built in a linear fashion, with features like pass-through cabinetry and interlock doors that allow only one door to be opened at a time, to minimize the risk of cross-contaminating or recontaminating a specimen.
Air handling and room finishes are critical to the success of molecular testing. A single speck of dust can carry bacteria that will contaminate a specimen. Seamless floors with cove bases; monolithic ceilings; and impervious, easily cleanable materials help keep extraction and amplification rooms free of potential contaminants. While the primary goal of typical BSL containment found in other lab testing areas is to protect the staff and environment from the specimens, the goal of molecular testing rooms is to protect the specimens themselves from contamination by the staff and environment.
Long-term success
Well-designed clinical labs provide ample space for maximum flexibility to enable labs to grow, change and adapt into the future. They also provide the infrastructure and layout needed to take advantage of the latest developments in technology and medicine. Sufficient power and data, robust heating and ventilation, careful design of engineering systems and the selection of appropriate materials and finishes are critical to the long-term success of clinical labs.
Andrew Jaeger is vice president of HKS Inc., based in the Detroit office. He can be reached at ajaeger@hksinc.com.
Automated testing systems and lab efficiency
Automated testing systems are being adopted throughout many areas of the hospital clinical lab. With traditional stand-alone analyzers, technicians tend to batch specimens until there are enough for a full load before placing specimens onto an analyzer, running a batch, then unloading the analyzer when testing is done. Stand-alone instrumentation often requires a high number of techs, as one tech can load, monitor and unload only a small number of analyzers at a time.
In an automated system, techs load individual specimens onto a robotic track in real time as the specimens arrive at the lab. The automated system handles everything from decapping and recapping tubes, aliquoting specimens and labeling the new tubes, delivering them to centrifuges as necessary, loading them onto the appropriate analyzers for testing, and automatically archiving them until they are ready to be disposed. Automated systems are able to retrieve a specimen days later and rerun it for additional testing, all without a tech’s ever having to touch the specimen.
Chemistry and immunology have been automated for well over 15 years. Beckman Coulter Inc., Brea, Calif., was an early, dominant manufacturer in the United States; Malvern, Pa.-based Siemens Healthcare and Swiss-based Roche are fast advancing in the chemistry and immunology areas. Automated technology started gaining popularity for hematology testing about 10 years ago, with Beckman Coulter and Japan’s Sysmex Corp. as prominent leaders in the field.
In the past few years, automated equipment and processes have been developed for microbiology, which, for the past century, has been a purely manual, time-consuming process. In manual microbiology, a tech smears a bacterial or fungal culture on a plate of growth media, incubates it for 24 to 72 hours, and visually inspects and manually counts any colonies that have formed. Then, a tech manually drops a grid of antibiotics on the plate, covers it, and puts it back in the incubator for another 24 to 72 hours, then inspects the plate again to see if any of the antibiotic spots have proved effective. It may take five or six days to determine, for example, that a blood infection is caused by penicillin-responsive bacteria.
With a fully automated system, the specimen is placed into a controlled environment where it is processed and sent via a track to a smart incubator, which scans the specimen at regular intervals. Colony-forming growths are automatically recorded in digital images that a microbiology tech can view on a computer monitor. The tech can flag anything of note, causing the smart incubator to send the plate in question to an automated susceptibility and identification analyzer. As with other automated testing systems, techs handle specimens only to load them into the front end of the equipment and dispose of them when done. Automated microbiology systems are scalable. As volumes grow, a lab may choose to add modules for automated detection or automated specimen archive and retrieval.
The entire industry is getting much closer to even more consolidated automation by adding hematology and coagulation testing to the chemistry and immunology lines. There are still a few last Food and Drug Administration hurdles to get over, but approval probably is coming within the next year or so.
By automating repetitive processes, hospital clinical labs can make the most of the intellectual capital of highly skilled, highly trained techs. Instead of spending their time preparing specimens or slides — mechanical duties that can consume their work days — they are able to do what they do best, which is use their cognitive problem-solving skills to analyze results.